Dr. I. Heertje studied the microstructure of food and detergent products for 25 years at the Unilever Research Laboratory in Vlaardingen, the Netherlands, lately as senior scientist. In the foods area his main interests were proteins and protein gelation, carbohydrates, dairy products, emulsions, and bakery and fat products. In this area, he published about over 20 scientific papers in the open literature and lectured at many conferences and institutions throughout the world. He was the former editor of the journal Food Structure for Europe and is now retired. In the last part of his scientific career he explored, in particular, the use of liquid crystalline phases in the structuring of food products. From his wide gallery of micrographs, he is contributing images of fat crystals and fat spreads, like margarine and butter. His three contributions to the Guest Food Microscopist sections were earlier available at the distans.lth.se:2080/ server but they have now been included in this Guest Food Microscopists 2 section.
|
Figure 1. Crystalline aggregate of needle-shaped and plate-like crystals, deposited on a substrate, in a margarine observed by TEM after replication. |
Fat is an indispensable part of our nutrition. It is a main energy source for the body. It is consumed in large amounts as margarines and butter, and is used for baking and frying and as spreads. On eating those types of products, we probably do not realize that we are eating crystals (Figure 1). But indeed in fat spreads the fat molecules of high-melting fats are crystallized in a regular arrangement into solid crystals. The type and the size of the crystals and their mode of interaction vary depending on the source of the fat blend and the processing conditions.
|
Figure 2. Fat crystalline network in a shortening observed by Cryo-Scanning EM. Careful inspection of the original micrograph reveals that the plates visible in this structure are in fact aggregates of single crystals. The open spaces in the structure indicate the areas where oil had originally been present before it was removed during the preparation of the sample for electron microscopy; oil removal was necessary to show the crystals..
|
Shortenings, frequently used in bakery applications, are composed of liquid oil and fat crystals only, whereas margarine and butter contain about 16% water in addition. Solid/liquid ratios of the fat phase in those products may vary widely, and are typically within the limits of 0.2 to 0.35 in margarine and butter at room temperature. Those products derive their consistency in particular from the organization of the fat crystals. An example of the product structure of a shortening is presented in Figure 2. The fat crystals form a plate-like three-dimensional crystalline network, with crystal bridges. The plates are in fact aggregates of single crystals as shown in Figure 1.
|
Figure 3. Fat crystalline network (yellow) and a water droplet structure (blue) in margarine observed by Cryo- Scanning EM. The shell around the water droplet has a crystalline structure. Bar: 10 µm. For open spaces see the caption at Figure 2.
|
Margarine also derives its consistency from a fat crystal network. The most striking difference, on comparing these products, is the presence of water droplets in margarine (Figure 3). Water droplets of a few micrometers in diameter are formed during intensive mixing of fat and water phases during processing. Crystals orientate at the water droplet surface and stabilize the water droplet. The continuous fat matrix again shows an interconnected network structure composed of plate-like crystal aggregates. In margarine products for various applications (creaming-, cake- and puff pastry), the nature of the fat crystalline network will vary with respect to the size, the shape, and the aggregation of the fat crystals.
|
Figure 4. Fat structure in butter showing a discontinuous arrangement of fat globules (yellow arrow) and fat crystals (green arrow) observed by Cryo-Scanning EM. Bar: 10 µm. For open spaces see the caption at Figure 2.
|
Butter has a completely different microstructure: it shows a discontinuous structure of fat globules and a crystalline fat matrix (Figure 4). Butter is made by churning dairy cream.
The fat globules observed have remained intact through the churning process. Different amounts of globules and the interglobular fat phase may be observed depending on the ripening procedure of the cream and other processing conditions.
Many people prefer the taste and the mouthfeel of butter over margarine and not without reason there is a product on the market named: I Can't Believe It's Not Butter. Apparently, the differences in structure between margarine and butter are reflected in their functional properties. But, at the same time, establishing the relationship between structure and function offers the possibility to manipulate the structure in such a manner that desired functional properties can be obtained. This is, in fact, one of the main objectives for doing microstructural research.
Dr. Heertje's scientific publications on foods:
- HEERTJE I., BOSKAMP M.J., KLEEF F. van, GORTEMAKER F.H. 1981. The microstructure of processed cheese. Neth. Milk Dairy J. 35, 177-179.
- HEERTJE I., VISSER J., SMITS P. 1985. Structure formation in acid milk gels. Food Microstruct. 4, 267-277.
- HEERTJE I., KLEEF F. van.1986. Observations on the microstructure and rheology of ovalbumin gels. Food Microstruct. 5, 91-98.
- VISSER J., MINIHAN A., SMITS P., TJAN S.B., HEERTJE I. 1986. Effects of pH and temperature on the milk salt system. Neth. Milk Dairy J. 40, 351-368.
- HEERTJE I., LEUNIS M., ZEYL W.J.M. van, BERENDS E. 1987. Product morphology of fatty products. Food Microstruct. 6, 1-8.
- HEERTJE I., VLIST P van der, BLONK J.C.G., HENDRICKX H.A.C.M., BRAKENHOF G.J. 1987. Confocal scanning laser microscopy in food research: some observations. Food Microstruct. 6, 115-120.
- HEERTJE I., EENDENBURG J. van, CORNELISSEN J.M., JURIAANSE A.C. 1988. The effect of processing on some microstructural characteristics of fat spreads. Food Microstruct. 7, 189-193.
- JURIAANSE A.C., HEERTJE I. 1988. Microstructure of shortenings, margarine and butter - a review. Food Microstruct. 7, 181-188.
- HEERTJE I., NEDERLOF J., HENDRICKX H.A.C.M., LUCASSEN-REYNDERS E.H. 1990. The observation of the displacement of emulsifiers by confocal scanning laser microscopy. Food Struct. 9, 305-316.
- HEERTJE I., HENDRICKX H.A.C.M., KNOOPS A.J., ROIJERS E.C., TURKSMA H. 1991. Use of mesomorphic phases in food products. European patent: 0 558 523 B1
- HEERTJE I., 1993. Microstructural studies in fat research. Food Struct. 12, 77-94.
- HEERTJE I. 1993. Structure and function of food products: a review. Food Struct. 12, 343-364
- HEERTJE I., PÂQUES M. 1995. Advances in electron microscopy. In: New physico-chemical techniques for the characterization of complex food systems (Dickinson E., ed). Blackie Academic, London, New York.
- HEERTJE I., AALST H. van, BLONK J.C.G., DON A., NEDERLOF J., LUCASSEN-REYNDERS E.H. 1996. Observations on emulsifiers at the interface between oil and water by confocal scanning light microscopy. Food Sci. Technol. 29, 217-226.
- HEERTJE I., LEUNIS M. 1997. Measurement of shape and size of fat crystals by electron microscopy. Food Sci. Technol. 30, 141-146.
- HEERTJE I., ROIJERS E.C., HENDRICKX H.A.C.M. 1998. Liquid crystalline phases in the structuring of food products. Food Sci. Technol. 31, 387-396.
- HEERTJE I. 1998. Fat crystals, emulsifiers and liquid crystals. From structure to functionality. Polish J. Food Nutr. Sci. 7/48, 7-18 (S).
- HEERTJE I. 1997. Vet zonder vet. Natuur en Techniek, 65 (2), 30-40.
© I. Heertje 1998
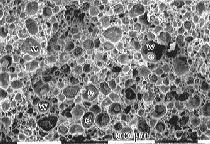 |
Figure 1. Structure of a 40% fat spread, containing 60% water observed by SEM. Water droplets (w) in a continuous fat matrix. Protein particles (e) in the water droplets. |
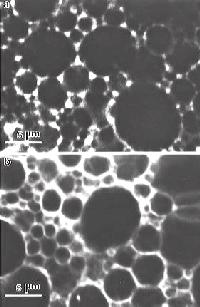 |
Figure 2. Structure of a 25% fat spread with 4% protein and 1% carbohydrates in the aqueous phase, observed by CSLM. Top: The fat, in between the droplets, was made visible by the fluorescer: Nile blue. Bottom: The protein, in particular at the interface of the droplets, was made visible by the fluorescer: fluorescein isothiocyanate. |
In the elaborate range of food products, fat spreads play an important part. In the earlier days, shortenings (100% fat), and margarines and butter (80% fat) set the scene. These products derive their consistency mainly from fat crystals and the way those crystals interact.
To decrease the proportion of fat in the diet of consumers in the Western world, spreads with 60, 40, 20 and even 0% fat have appeared on the market. With the decreasing fat content, the composition and the structuring of the aqueous phase becomes gradually more important for obtaining a good fat spread.
Low fat spreads (20% fat) have a structure comparable to that of margarine. In general, these products are water-in-oil emulsions, in which a continuous fat matrix surrounds the dispersed water droplets (Figures 1 and 2).
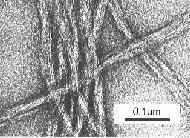 |
Figure 3. Fine stranded polysaccharide gel network of curdlan (Courtesy T. Harada) observed by TEM. |
Therefore, those products behave in many respects (mouthfeel, spreadability) similar to classical fat spreads. With even lower amounts of fat (<20%) it becomes impossible to keep the fat in the continuous phase. When water becomes the main component of the food system, we are faced with the problem to structure it while giving the product fat-like properties.
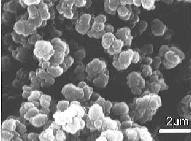 |
Figure 4. Globular particles in the formation of a particle gel of ovalbumin observed by SEM. |
Various strategies to achieve this have been developed. Large amounts of water can be enclosed in fine stranded networks of polymer chains, with many entanglements and junction zones.
Carbohydrates (carrageenan, pectin, curdlan (Figure 3) and proteins (gelatin) may show this behaviour. In other cases, in particular for proteins, coarse particle gels are formed by aggregation of macromolecular assemblies in globular form (e.g., yogurt) (Figure 4).
However, in the design of very low and zero fat spreads, these strategies fail to imitate the properties of margarine. This was accomplished by using liquid crystalline or mesomorphic phases.
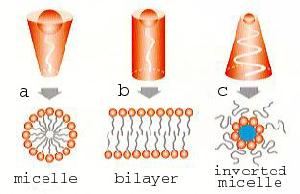 |
Figure 5. Aggregation behavior of emulsifier molecules (a) Head group larger than the tail: micelle formation in water (b) Cylinder-shaped molecule: formation of parallel bilayers (c) Head group smaller than the tail: formation of inverted micelles, enclosing water. |
|
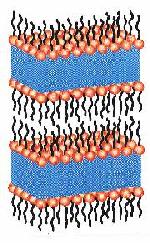 |
Figure 6. A schematic drawing of a lamellar phase. The emulsifier molecules are arranged in parallel sheets in such way that the hydrophilic head groups of the molecules are in contact with water and that the hydrophobic chains are in contact with each other and excluded from water. |
|
Such phases can be formed from emulsifiers such as monoglycerides (monoacylglycerols) and phospholipids. The molecules of these compounds are characterized by a hydrophilic water-attracting head and a hydrophobic water-rejecting tail and display a specific aggregation behavior that is determined by their geometry (Figure 5). The situation of the greatest interest in the structuring of food products is the one involving more or less cylindrically shaped molecules (Figure 5b). In that case, bilayer structures are formed, which may enclose water layers, which give rise to a so-called lamellar phase, a three-dimensional structure of alternating bilayers and water layers (Figure 6). The lamellar phase can be obtained by heating, e.g. monoglycerides, in the presence of water to above a certain transition temperature and can, under certain conditions, take up all the available water into a continuous swollen lamellar phase (Figure 7).
When this phase is cooled down below its transition temperature, the lamellar phase structure is retained and a highly viscous phase, the so-called a-gel phase is formed. By crystallisation this phase in turn may be transformed into an even stiffer gel type, the coagel.
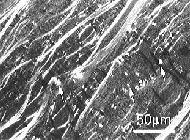 |
Figure 7. Monoglycerides (0.05g/g) and water heated above the transition temperature (60°C), observed by LM. Swollen streaky lamellar texture and some (partly swollen) multilamellar vesicles (arrows). |
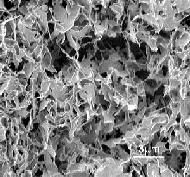 |
Figure 8. Network structure of monoglyceride (0.05g/g) crystals in a coagel observed by SEM. In the open space between the crystals water was originally present. |
It appears to be composed of a network of monoglyceride crystals enclosing water (Figure 8). Both gel types have much to offer in the structuring of food products. a-Gel can be applied in softer products and is optimal in aerated products because of its strong foaming capacity in contrast to the coagel. Coagel has been applied in the development of a "fat" free margarine for the North-American market. This product contains 3% of monoglyceride in a coagel state. The product shows a remarkably fatty impression. This may well be related to the striking resemblance of the network of monoglyceride crystals (Figure 8) with the network of triglyceride crystals in a shortening or an 80% fat spread such as margarine. Apparently, the enclosed liquid, i.e., oil in the case of high fat products and water in a "fat" free margarine, is of minor importance for the perceived fatty impression. The structure of the network and the function of the product appear to be clearly related. Undoubtedly, the further exploration of the relation between structure and function will open up new roads in the design of new food products.
References
KROG, N., BORUP A.P. 1973. Swelling behaviour of lamellar phases of saturated monoglycerides in aqueous systems. Journal of the Science of Food and Agriculture 24, 691-701.
HEERTJE I., HENDRICKX H.A.C.M., KNOOPS A.J., ROIJERS E.C., TURKSMA H. 1991. Use of mesomorphic phases in food products. European patent 0 558 523 B1
HEERTJE I., ROIJERS E.C., HENDRICKX H.A.C.M. 1998. Liquid crystalline phases in the structuring of food products. Food Science and Technology 31, 387-396.
© I. Heertje 1999
How do you like your eggs in the morning?
Some not fully respected people may have experienced that the content of raw eggs is quite different from a nice hard-boiled or scrambled egg consumed at the breakfast table. Those of us, who prepare their own food, know that an egg consists of 3 parts: the shell, the eggwhite, and the yolk. Only the latter two are used as a food. It is easy to observe how the eggwhite and the yolk solidify during frying. The solidification actually is a complex process consisting of a sequence of structural changes. Macroscopically, the translucent viscous mass turns white and solid.
The hardening is due to ovalbumin, which is the main eggwhite protein. When an aqueous solution of this protein (20g/100g) is heated, it forms an irreversible gel. The gel formation can be followed by measuring the shear modulus (some measure of the hardness) as a function of temperature. It appears that at about 75°C the hardness increases dramatically up to about 90°C. On subsequent cooling and reheating, the hardness is not much affected. This in contrast to gelatin, another well-known protein, which, after gel formation, dissolves again on heating.
Proteins play an important role in many food systems. Food technologists, who understand structure formation and relationships between macroscopic
properties microstructure and molecular properties, may fruitfully apply this information in the development and the processing of food products.
In this context, ovalbumin gels, prepared by heating at 100°C under different conditions of pH were examined by scanning and transmission electron microscopy and rheologically characterized. The latter was performed by stretching a small sheet of the gel and measuring the elongation as a function of the applied force. In addition, changes in the conformation of the protein molecules were examined. This was accomplished by nuclear magnetic resonance (NMR) measurements.
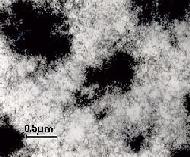 |
Fig. 1. A heat-set ovalbumin gel at pH 5. Dark area represents protein. Note an aggregated inhomogeneous protein structure. Thin-sectioning TEM. Bar: 0.5 µm |
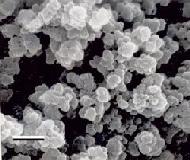 |
Fig. 2. A heat-set ovalbumin gel at pH 5. Note the granular structure. SEM after freeze-drying. Bar: 2 µm |
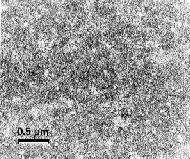 |
Fig. 3. A heat-set ovalbumin gel at pH 10. Dark area represents protein. Note the homogeneous distribution of protein filaments. Thin-sectioning TEM. Bar: 0.5 µm |
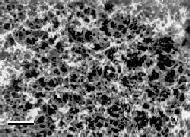 |
Fig. 4. A heat set ovalbumin gel at pH 10. SEM after freeze-drying. The cellular structure is induced by ice crystals formed during sample preparation. Bar: 2 µm
|
Using this technique it is possible to study the behaviour of the protein molecules at the different conditions of pH and temperature. In particular, information is obtained on the degree of unfolding of the
protein molecules, which is important to understand the aggregation behaviour
of the protein molecules.
An ovalbumin gel prepared at pH 5, shows an inhomogeneous distribution of strongly aggregated protein particles (Figs. 1 and 2), whereas a homogeneous distribution of fine protein strands is observed at pH 10 (Figs. 3 and 4). This behavior is ascribed to the molecular properties of the proteins. At the low pH, close to the isoelectric point of the ovalbumin at pH 4.5, strong hydrogen bonds, ionic- and strong hydrophobic interactions occur between the uncharged protein molecules. They result in the formation of dense protein aggregates. In contrast at high pH, under influence of electrostatic repulsion, protein molecules unfold and are irreversibly cross-linked mainly via covalent disulfide bonds without much further interactions. For this reason, gels prepared at such a high pH will not be affected by subsequently lowering the pH.
The differences are further reflected in the mechanical and other properties of the gels. A structure, which is composed of flexible, unfolded, but not strongly interacting protein chains is much more extensible than the aggregated structure composed of compact, strongly interacting protein molecules. In the latter structure, regions of low protein concentration tend to act as weak points, resulting in a gel, which breaks easily. This
also explains why gels at pH 5 are opaque and easily release water, whereas pH 10 gels are transparent and do not loose water.
Combining this type of knowledge on all levels of structural organization is of great help in the optimal use of proteins and modified proteins in foods and food processing.
© I. Heertje 2001
|